Writing in the journal of frontiers by a team of Spanish.
Soccer is one of the most popular team-based sports worldwide for audiences, attendance, and participation. Official matches consist of two halves of 45-minutes each, interspersed by a 15-minute break.
Successful soccer performance depends on multiple, complex, and interdependent factors, including anthropometric traits, maximal speed, change-of-direction ability (COD), and aerobic and anaerobic capacities (Stolen et al., 2005; Hulse et al., 2012). Soccer is an intermittent high-intensity sport, which includes various types of running with rapid changes of directions, starts, stops, jumps, and kicks (Alfredson et al., 1996).
Therefore, physical performance is commonly assessed and monitored as the outcome of standardized motor tasks requiring maximal speed, COD ability, balance, flexibility, explosive strength (Malina et al., 2004), local muscular endurance, and static muscular strength, particularly for long-term athletic training (Carling, 2013).
The energy demand in soccer players is mainly dependent on aerobic capacity (Stolen et al., 2005). Adult soccer players usually cover between ∼10 and 12 km, while in young soccer players the distances covered during competitions are lower: 6.5, 7.4, and 8.1 km (for under −13, −14, and −15, respectively) (Buchheit et al., 2010).
Moreover, higher levels of aerobic capacity enhance recovery from high-intensity interval loads (Svensson and Drust, 2005). This capacity also seems to be a prerequisite to improving the efficiency of anaerobic capacity when performing high-intensity intermittent efforts (Tomlin and Wenger, 2001). Also, when performing repetitive sprints tests, the best players showed more tolerance to fatigue (Reilly et al., 2000b). Finally, change-of-direction ability is also able to discriminate recreational and non-soccer players matched for intermittent endurance capacity (Coratella et al., 2016).

Maximal speed and COD ability tests are commonly used in talent identification programs (Rommers et al., 2019), as during the match activities involving accelerations (ACC), maximal speed, and COD ability number in the range of 150–250 n (Bangsbo et al., 2007). Although the physiological measures (e.g., absolute maximal oxygen uptake—VO2max) were generally more discriminative than anthropometry (Reilly et al., 2000a), positive correlations between body mass, fat-free mass, and skeletal muscle mass have been observed (Boraczyński et al., 2015).
Indeed, the fat-free mass has been shown to be a significant predictor of maximal speed, endurance, and jump capacity (Gil et al., 2014). Nevertheless, performance improvements are also dependent on increases in anthropometric traits, body mass, skeletal muscle mass, heart and lung mass, hemoglobin level, and blood volume as well as the maturation of the nervous system (Stolen et al., 2005). This highlights the importance/influence of growth and maturity in performance. The optimal period (window of opportunity) to improve physical, technical, and physiological capacities has been postulated to occur between the ages of 12 and 16 years, during maturation (Lloyd and Oliver, 2012).

During adolescence, the interaction between genes, hormones, nutrients, and environmental factors triggers a series of physical and functional alterations in the body (Borges et al., 2018). The capacities mentioned above show their greatest improvement during the adolescent growth spurt (Pearson et al., 2006). This period does not occur at the same time in all players, favoring the early maturing players.
Teixeira et al. (2014) showed that early maturing players are taller and heavier and attained higher absolute values in ventilatory thresholds and the maximal peak of oxygen consumption compared to average maturing players, even after normalizing for interindividual variability in anthropometric traits. Because of their advanced anthropometric and physical profile (Figueiredo et al., 2010; Meylan et al., 2010), early maturing footballers play regularly and are more often selected for regional and national teams (Meylan et al., 2010).
The literature has confirmed that players that are born in the first months of the year, normally have an advantage as their maturation is more advanced compared to athletes who were born in the third and fourth quarters of the year (Deprez et al., 2012). In a study that analyzed age and maturity in soccer, Rommers et al. (2019), showed significant differences among several successive age categories, highlighting that the pubertal period is a critical time for skill acquisition and development of performance in youth elite soccer players.
Following the Long-Term Athlete Development model, players aged 16–18 are in the “training to compete” phase of development with highly structured training (Ford et al., 2011). Until that phase, players are still developing their metabolic conditioning, endurance strength, power, and cognitive skills specific to soccer. Therefore, their response to training load and recovery will be different from adults, who train predominantly to maintain and improve physical traits and performance, as they already have more developed physical characteristics (Lloyd and Oliver, 2012).
Thus, to ascertain appropriate loads for each player also regarding their age, it is important to monitor training loads individually (Vahia et al., 2019). Monitoring the training load in soccer is a key component of the training process as it helps set an adequate balance between training and recovery (Gaudino et al., 2015).
Author: Hadi Nobari et all
Published online: https://www.frontiersin.org/articles/10.3389/fphys.2020.597697/full
“Analysis of Fitness Status Variations of Under-16 Soccer Players Over a Season and Their Relationships With Maturational Status and Training Load”
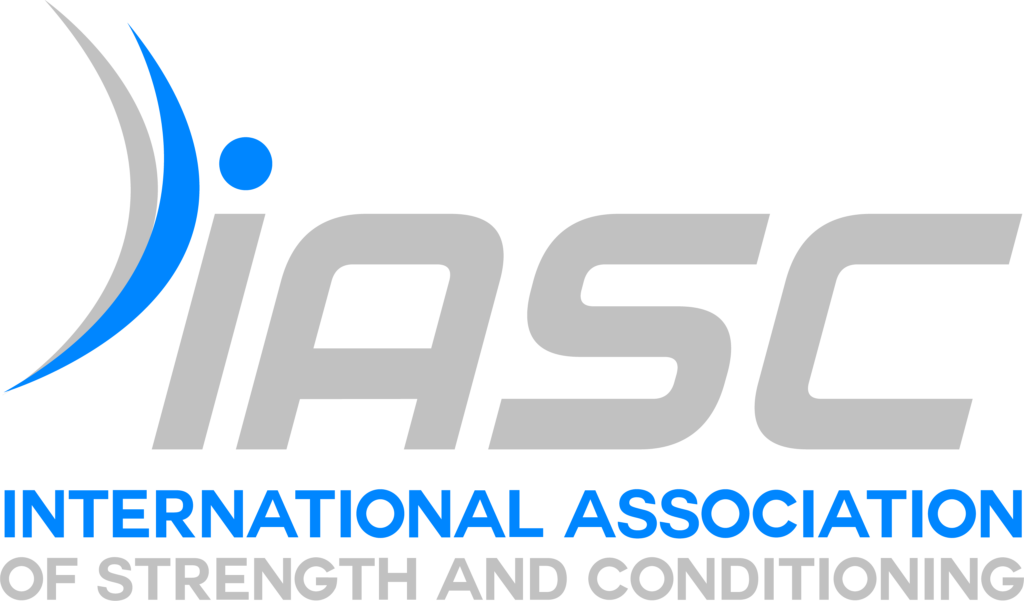
Stay updated with the International Association of Strength and Conditioning